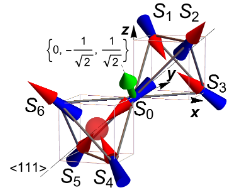
In certain materials called "spin ices" magnetic atoms interact strongly with each other to form composite objects known as “magnetic monopoles”. These are quasi-particles behaving similarly to a “North” pole of a magnet that has broken free from its “South” counterpart. There is a lot of experimental evidence for the existence of these exotic objects, which were proposed in 2008, but their motion mechanism has remained quite mysterious. The “hop” of a monopole from one site in the crystal lattice to the next can be achieved by flipping the direction of a single magnetic moment, however at low temperatures the magnetic moments do not have enough energy to do this. How come, then, that experiments show monopoles happily hopping about at a rate of between 1 hope per microsecond and one hope per millisecond, even at extremely low temperatures? A new paper proposes a hopping mechanism based on quantum physics: as a monopole arrives in a lattice site, it induces changes in the fields acting on the atomic magnetic moments surrounding it which enable them to "tunnel" through the energy barrier. The authors obtain theoretical estimates of this tunnelling rate and they find it to be in broad agreement with the fastest rates observed in experiment. They also discuss how interaction with the environment ("decoherence") can slow down the monopoles thorugh a phenomenon known as the "quantum Zeno effect". A better understanding of monopole motion in spin ice materials may enable future technologies based on moving mangetic monopoles, rather than electric charges.
A magnet has a North and a South pole. Break a magnet in two, and you get two new magnets, each with its own North and South poles. No matter how small you make a magnet, it always has two poles – a “dipole”. In fact, despite extensive searches, no one has ever observed an isolated North or South pole – a “magnetic monopole”.
On the other hand, physicists have known for some time that certain magnetic materials, called “spin ices”, host quasi-particles that effectively behave like magnetic monopoles. If you look closely, the quasi-particle is actually formed of individual, atomic-scale dipoles (the blue and red arrows in the figure) – but from further apart the collective motion of the dipoles is best described in terms of magnetic monopoles moving within the crystal (the red sphere). This was put forward as a theoretical idea by Castelnovo, Moessner and Sondhi in 2008 and since then lots of experimental evidence has accumulated that supports it.
One enduring mystery, however, is how the monopoles manage to keep moving about, even at very low temperatures. To understand why this is a mystery consider again the figure. For a monopole to move, a dipole has to flip – for example, in the figure there is a monopole in the bottom-left corner tetrahedron (because there are more dipoles pointing into the tetrahedron than out of it) but there is no monopole in the other tetrahedron (because the number of dipoles pointing into it and out of it is balanced). To move the monopole, one could flip the dipole sitting at the corner shared by the two tetrahedra. After this, the balance would be restored in the bottom-left tetrahedron, and the monopole would sit in the top-right one. The problem with this is that at very low temperatures the dipoles do not have the energy that would be required to flip in this way – yet, there is plenty of evidence that the monopoles keep moving at very low temperature. The characteristic “hopping rate”, for the most common spin ice materials, ranges from of the order a one hop per microsecond to one hop per millisecond, depending on the type of experiment being performed.
In a recent preprint we present evidence that this mysterious low-temperature hopping is achieved through quantum tunnelling: a phenomenon that allows a quantum object to overcome an obstacle which would, according to the classical laws of physics, require more energy than the system has available to it. We show that the magnetic dipoles forming a monopole experience fields that are transverse to the dipoles themselves, which in turn induce the tunnelling. We compute the monopole hopping rates resulting from this scenario and find them to be broadly consistent with available observations. Finally, we discuss how interaction with the environment, modelled as a set of random observations, can slow down the process through the so-called “quantum Zeno effect” where frequent observations of a quantum system collapse its wave function back to the initial state.
Our paper has recently been accepted for publication in Physical Review Letters.